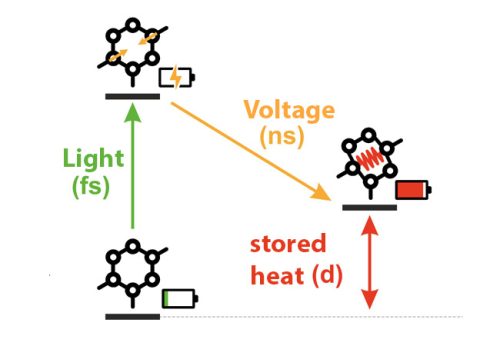
In the future, our economy will use more and more renewable energy and therefore effective, high-capacity energy storage technologies will be essential – for example a completely new technology like molecular solar thermal (MOST) energy storage. This is a promising technology for storing solar energy, which is still in its infancy – a kind of heat battery comprising a large number of synthetically created molecules that together store energy from the sun. They are currently being investigated in the interdisciplinary project FORMOST.
The molecules undergo structural changes on contact with sunlight. Chemical bonds are broken, formed or twisted around. As these processes are triggered by light, they are called photo-induced reactions. Their special feature is that under the influence of light, the molecules absorb energy by flipping into an excited state. The energy can be released again later, as the molecule returns to its original state. So it is a type of on-off switching, and because light triggers the mechanism, the molecules are called photoswitches. Three such photoswitches created in the laboratory are under investigation at FORMOST: norbornadienes, azaborines and azobenzenes. When exposed to light, all three switch from their ground state to a higher-energy storage state, thus changing their molecular structure and in some cases also their color.
Take norbornadienes for instance – aromatic hydrocarbons containing seven carbon atoms. When stimulated by light, two new bonds are created between certain atoms. This results in a number of three or four-membered rings. The molecule bends like a tension spring – and this is how it stores energy. The energy in the “spring” can be released later as heat. This takes place slowly when uncontrolled, but can also be regulated. Azaborines are a relatively new type of photoswitch and they, too, create a new bond. A relaxed six-membered ring absorbs energy by forming two four-membered rings. It is relatively easy to use them as energy storage molecules. The reaction is somewhat different with azobenzenes, which twist like a screw to enter the excited state.
Resource-saving approach
MOST molecules have several advantages. First, they consist largely of carbon, oxygen, nitrogen and hydrogen. This means they consume less in terms of resources, needing neither rare earths nor heavy metals nor plastic. Furthermore, MOST is based on a simple process. “All the steps – conversion, storage and release of energy – are united in a single molecule,” says Josef Wachtveitl, head of the Wachtveitl group that is involved in FORMOST and works on ultrafast spectroscopy at Goethe University’s Institute for Physical and Theoretical Chemistry.
As the heat is stored directly, there are no conversion processes like turning electricity into heat. This means that the losses that otherwise occur during any energy conversion are totally absent – which is another benefit. Direct comparison of MOST with conventional solar heat storage systems reveals that these molecules produce far better results. Conventional solar thermal systems use black collectors to absorb heat during the daytime, which is then stored as hot water. That process can function only at very limited times, and it is uncontrolled because heated water can release the heat spontaneously. By contrast,. MOST molecules have to be activated to give up their heat – by a catalyst, light of a different wavelength or heat. The energy is released on demand, at a chosen time – like flicking a switch. The heat can also be stored for much longer than with solar thermal systems, i.e. for weeks or even months. Then there is control over the location: the long, controllable storage period renders the storage systems easily transportable. What is more, the intensity can also be regulated by changing the quantity of added activator. “MOST gives us much more flexibility for storing solar heat,” according to Wachtveitl.
However, the molecules must possess four characteristics to be useful as heat stores. First, they should be able to absorb a large proportion of natural sunlight. At present the molecules tend to absorb only in the UV range where it borders on visible light, i.e. at wavelengths from 200 to 400 nanometers. This light is indeed high in energy, but in the solar spectrum it has a very low intensity. The maximum intensity of sunlight is in the green range, at wavelengths of between 500 and 600 nanometers. “This is exactly the area we’re working on and we’re trying to modify the photoswitches so that they absorb in this range – that’s our most important goal,” explains Jonas Lienert, who is a member of the Wachtveitl group and also works on the FORMOST project. Second, the molecules should hold a lot of heat, and so the difference in the energy levels between the ground state and the excited storage state should be as large as possible. Third, the molecules must not discharge spontaneously, with the storage state lasting for as long as possible while remaining stable and controllable. And the fourth criterion is that the heat storage systems should be as small and lightweight as possible, but efficient at the same time. In the best case, every light particle (photon) should trigger the reaction that flips a molecule into the energized storage state. “Then we would have maximum efficiency.” In the project, the molecules are being modified to achieve the best properties in as many of the criteria as possible. At the moment none of them are perfect. Norbornadienes, for example, exhibit a large energetic difference between the ground state and the excited state, but they absorb only a small proportion of sunlight.
Groups from several German universities are participating in the project. They all have special tasks and over the next few years they will combine their findings to build up an overall picture. Wachtveitl’s group is looking more closely at the superfast charging processes in photoswitches. To find out what is going on in the molecule, they need resolution in the femtosecond range. A femtosecond is 10-15 seconds. This is hard to imagine, but it can be illustrated by the following comparison: light takes roughly one second to travel from the earth to the moon. In one femtosecond it would cover only the tiny distance of 0.3 micrometers (0.0003 millimeters).

Wachtveitl’s group works with the pump-probe technique. This method is used to achieve resolution of dynamic photo-induced processes. It allows investigation of many things such as the influence of light on leaf pigments like chlorophyll, or on the receptors in the human eye – or the ultrafast switching from the ground state to the excited state in MOST molecules. In the pump-probe technique, a semi-transparent mirror divides a laser beam into two beams, or laser pulses. The first one (pump) excites the molecules, and the second one probes them. The trick is that there is a time delay between the pump pulse and the probe pulse. Pictures are taken of the molecule at longer and longer intervals, and at the end they are collated to create a “film,” like a flip book. “By making molecular films with time-resolution of just a few femtoseconds between the individual pictures, we can see exactly what is going on in the molecule after photoexcitation,” Lienert explains. Here it is important that the probe-pulse consists of white light, because white light contains all the colors of the spectrum – so the individual photos will show everything that happens in the molecule. The discharge process triggered by the catalyst, light or heat can be observed in the same way. As this process is a lot slower, it is easier to record. Wachtveitl and Lienert want to discover how well the molecules convert light into energy. What undesired side reactions take place? How can efficiency be improved? What is the maximum duration of storage? “This gives us a better understanding of the effects of the molecular structure on the four desired characteristics,” Wachtveitl explains. “Then we can give our colleagues working on synthesis at FORMOST pointers for how the molecular structure could be adapted to improve the properties of the molecules.”
Initial attempts at application
Where is this basic research leading? MOST applications are already being tried out. For example, the molecules are placed on glass surfaces. The glass is intended to be made into windows later on, which can store energy during the day and release it again at night. MOST molecules that can be pumped within a closed cycle are also already in development. They could be installed as panels on roofs, where the sun will induce them to switch to their storage state. The heat stores could be kept for a long time using a large tank, and activated as required. In general, MOST storage systems could be used anywhere that needs to be heated, such as in cars, houses, factories and housing for farm animals.
“Every alternative to oil and gas is useful and contributes to a more climate-friendly form of heating,” says Wachtveitl. MOST systems are very promising, he adds. Once they have been established, they can operate for a long time and their processes for storing, converting and releasing energy are completely CO2-neutral. And one of their secondary effects is that MOST heat storage systems are decentralized and can be set up anywhere, because all the processes occur inside a single molecule – so large production plants are not required.
Andreas Lorenz-Meyer
The FORMOST project is a collaboration between Goethe University and the universities in Tübingen, Giessen, Heidelberg and Erlangen-Nuremberg. Wachtveitl’s group researches the molecular mechanisms of energy storage and release in MOST molecules. Other groups are investigating molecule synthesis and modelling. The project will run from 2023 to 2027, receiving a total of around 4.8 million euros in funding from the German Research Foundation (DFG).
MOST molecules release stored energy under the influence of catalysts, light of other wavelengths, or heat. In the case of heat as the initiator, one idea is to start a chain reaction in which the heat energy released by a few molecules will cause more and more energy to be released from the other molecules faster and faster – a process called autocatalysis.
FORMOST on YouTube
The FORMOST research group now has its own YouTube channel, which will offer exciting insights into this research, and also into topics surrounding research and the university.
www.youtube.com/@YTFORMOST5499