Biophysicist Achilleas Frangakis uses cryo-electron tomography to study the inner life of dangerous bacteria.

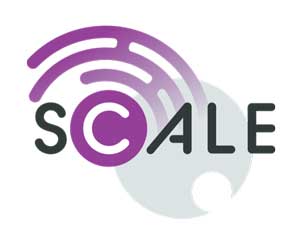
Acinetobacter baumannii is the name of one of the most dangerous hospital germs, which often attacks people with weakened immune systems. The bacterium has built up resistance to many antibiotics, something it was able to do because it possesses efflux pumps, whose jobs include pumping antibiotics out of the cell – which helps in developing resistances. The adjacent image of the cell envelope of Acinetobacter baumannii provides a detailed depiction of its interior. The pumps, whose shape is reminiscent of corks for wine bottles, are shown in orange. Other structures are also easy to recognize: ribosomes are shown in yellow, adhesion proteins in purple, membrane proteins in blue, and membranes in white. “We created this 3-D reconstruction using cryo-electron tomography,” explains Achilleas Frangakis from Goethe University Frankfurt’s Institute for Biophysics. “It shows how much detail and complexity can be presented with this imaging procedure.” Cryo-electron tomography (cryo-ET for short) is used to show individual cells, tissue segments or organelles at a resolution in the sub-nanometer range. It does so by combining a large number of individual 2-D images of freeze-dried samples into a 3-D image. Unlike other methods, including single-particle cryo-electron microscopy or x-ray crystallography, cryo-ET does not require the proteins to be isolated or purified in advance. “With cryo-ET, everything remains unchanged, enabling us to visualize the molecular architecture of an individual protein – while it’s working.”
The snapshots of the cell’s interior are very useful to scientists. Frangakis’ work focuses on cell-cell contacts, i.e. those regions where individual cells interact with their neighboring cells. “There are two reasons why it’s difficult to create images of them: the small size of the protein building blocks, and their flexibility – which they need, among others, to promote quick and effective wound healing. So we’re dealing with very small, very dynamic structures. The challenge lies in achieving atomic resolution using quasi-living samples that naturally adopt different shapes.”
Bacteria also have cell-cell contacts, and they are what Frangakis is focusing on. His “pets” are mycoplasma bacteria, which cause infections in the respiratory or genital tracts. These pathogens do not have cell walls, which constitutes a problem, since many antibiotics kill bacteria by attacking the cell wall. Using cryo-ET, the scientist has already been able to identify the essential protein P116. “It’s responsible for supplying the mycoplasmas with the necessary cholesterol and lipids that they extract from the infected host cells.” At present Frangakis is testing P116 as a possible means of transporting into human cells hydrophobic pharmaceutical agents, which until now have been difficult to transfer to their site of action. He is also working with the Franco-German EFFORT consortium in another project to develop inhibitors for the efflux pumps many bacteria – not just Acinetobacter baumannii – use to keep antibiotics at bay.
What does Frangakis want the SCALE project to achieve? “Structural cell biology addresses an extremely complex network of dynamic interactions. To analyze them, we’re developing a digital twin of the real subcellular structures.” Their behavior can then be simulated virtually and analyzed using machine learning. Frangakis’ hope is that “the twin will revolutionize our understanding of the interactions between the cells.” He appreciates SCALE’s interdisciplinary approach, which he says will advance research into the quantitative description of subcellular architectures. He also commends the “comprehensive support” the project is receiving both from the boards of the two participating Max Planck Institutes and from Goethe University Frankfurt’s Faculty of Biochemistry, Chemistry and Pharmacy as well as its Faculty of Biological Sciences.
The cell’s architecture
What do the building blocks of life look like, and how do cellular players interact with one another? As part of the excellence cluster initiative SCALE (Subcellular Architecture of Life), Frankfurt scientists have set out to explore these important questions. The interdisciplinary project brings together several research groups that focus on biophysics. Biophysicist Achilleas Frangakis and bioinformatician Kathi Zarnack are part of the team, and pursuing great goals.
Link: SCALE
Recognizing links and connections: bioinformatician Kathi Zarnack analyzes cells using a multi-omics approach.
Protein biosynthesis, i.e. the process of building a protein based on a gene, has two phases. During transcription, a single-strand copy, the mRNA, is made from the double-strand DNA. The mRNA transports the genetic information from the cell nucleus to the ribosomes in the cytoplasm, where the stored information is translated into a protein. “But the mRNA is more than just a messenger molecule,” says bioinformatician Kathi Zarnack, head of the Computational RNA Biology research group at the Buchmann Institute for Molecular Life Sciences. An incredible amount of regulation takes place on the RNA, and important decisions are made there. One key player is the RNA binding proteins. “They are present the entire time. During the copying process they hitch a ride on the transcription machinery and then jump directly onto the RNA when it emerges from the polymerase. This is when they decide what the RNA will do, where it goes inside the cell, how stable it is, and which function it performs.”
If these allrounders do not function properly, diseases such as cancer or neurodegenerative diseases like Alzheimer’s and ALS can be the result. That is why it is all the more important to decode the regulatory mechanisms in which the RNA binding proteins are involved. Herein lies the focus of Zarnack’s research. Her approach of analyzing data is called multi-omics analysis, as part of which the entire pool of each type of molecule is measured: in proteomics all the proteins in the cell, in transcriptomics all RNA molecules, and so on. The measurements of the various molecule types are then linked together to decode their interactions.
Zarnack explains the advantage of the approach using the spliceosome, a gigantic piece of machinery consisting of 200 to 300 proteins in the cell. Its job: make the RNA molecules fit for translation. Whereas initially, they still have some non-coding regions, during splicing, these parts are excised and the coding regions subsequently stuck back together. This yields mature RNA, which will later code the protein. Central to the spliceosome machinery is the component carrying out the splicing reaction. If this component is genetically modified, it can make a person sick. “Whereas we might expect that all the body’s cells are affected in equal measure if this central core of the spliceosome were damaged, that is not the case. Instead, the changes in the spliceosome lead to a specific eye disease known as macular degeneration, because it’s primarily retina cells that react to that.” Why only these cells and not others as well?
High-resolution single molecule microscopy cannot provide the answer. “We can see how an individual protein binds. The fine detail is incredible, but we only see a single regulatory event.” There’s no overview. This is exactly where multi-omics come into play. “We use transcriptome analysis to investigate all the splicing events at once. This shows what’s changing in retina cells – and how these changes differ from those in other cells in the body. We wouldn’t be able to understand that if we were looking at the binding of the protein to a single RNA.”
Zarnack also applies this systemic approach in SCALE. To her, multi-omics analyses are a supplement to high-resolution microscopy. “Whereas the latter supplies the detailed knowledge, multi-omics provide the overall picture – which we can use to recognize links and connections, i.e. what happens in the whole cell when something changes at just one place. From this systemic viewpoint we can then switch back to high-resolution microscopy to see what the altered molecules are doing and how they bind to one another. It’s all part of an alternating exchange of information – which we in SCALE want to use to clarify basic molecular mechanisms so that one day we will be able to predict disruptions in RNA regulation.”
Andreas Lorenz-Meyer
ExStra – The Excellence Strategy of the German Federal and State Governments‘
Goethe University Frankfurt is applying for the upcoming round of the Excellence Strategy of the German federal and state governments with four new clusters on the following research topics: Trust in conflict (ConTrust), infection and inflammation (EMTHERA), the origin of heavy chemical elements (ELEMENTS), and cellular architectures (SCALE). These applications bring together the competencies and pioneering ideas of Goethe University Frankfurt with those of colleagues in the Rhine-Main Universities (RMU) alliance and additional partners from four major non-university research organizations. The Cluster of Excellence “Cardio-Pulmonary Institute” (CPI), first set up in 2019, will submit a full proposal next year.